Thank you for visiting nature.com. You are using a browser version with limited support for CSS. To obtain the best experience, we recommend you use a more up to date browser (or turn off compatibility mode in Internet Explorer). In the meantime, to ensure continued support, we are displaying the site without styles and JavaScript.
Scientific Reports volume 13, Article number: 15153 (2023 ) Cite this article Benoxacor

The widespread use of synthetic pesticides has resulted in a number of issues, including a rise in insecticide-resistant organisms, environmental degradation, and a hazard to human health. As a result, new microbial derived insecticides that are safe for human health and the environment are urgently needed. In this study, rhamnolipid biosurfactants produced from Enterobacter cloacae SJ2 was used to evaluate the toxicity towards mosquito larvae (Culex quinquefasciatus) and termites (Odontotermes obesus). Results showed dose dependent mortality rate was observed between the treatments. The 48 h LC50 (median lethal concentration) values of the biosurfactant were determined for termite and mosquito larvae following the non-linear regression curve fit method. Results showed larvicidal activity and anti-termite activity of biosurfactants with 48 h LC50 value (95% confidence interval) of 26.49 mg/L (25.40 to 27.57) and 33.43 mg/L (31.09 to 35.68), respectively. According to a histopathological investigation, the biosurfactant treatment caused substantial tissue damage in cellular organelles of larvae and termites. The findings of this study suggest that the microbial biosurfactant produced by E. cloacae SJ2 is an excellent and potentially effective agent for controlling Cx. quinquefasciatus and O. obesus.
Domnic Colvin, Vishnu Dhuri, … Avinash Kale
S. Appalasamy, MH Alia Diyana, … JG Boon
Antonia Pott, Mirco Bundschuh, … Ralf Schulz
Tropical nations are home to a disproportionate number of illnesses transmitted by mosquitoes1. The relevance of mosquito-borne diseases is widespread. More than 400,000 people die each year from malaria, and serious illness epidemics from dengue, yellow fever, chikungunya, and zika have been reported in several metropolitan areas2. There is an association between vector-borne illnesses and one-sixth of all infections globally, with the most significant occurrence being tied to mosquitoes3, 4. Culex, Anopheles, and Aedes are the three mosquito genera most frequently implicated in the transmission of illness5. Dengue fever is an infection spread by the vector Aedes aegypti mosquito6, and its prevalence has been on the rise over the last ten years, making it a significant threat to public health4, 7, 8. According to the World Health Organisation (WHO), more than 40% of the world’s population is at risk of developing dengue fever, and 50–100 million new infections occur each year in over 100 countries9,10,11. Dengue has become a significant public health problem since its incidence has increased globally12,13,14. Anopheles gambiae Giles, often known as the African malaria mosquito, is the most important vector of human malaria in tropical and subtropical areas15. The West Nile Virus, St. Louis encephalitis, Japanese encephalitis, and viral infections of horses and birds are all spread by the Culex, often known as common home mosquitoes. Aside from that, they are known to spread bacterial and parasite diseases16. There are more than 3000 species of termites around the globe, and they have been there for over 150 million years17. Most pest species live in the soil and eat wood and wood products that contain cellulose. A prominent pest, Indian white termite, Odontotermes obesus causes significant damage to essential agricultural crops and forest plantation trees18. In agricultural regions, termites inflict tremendous economic losses to various crop plants, tree species, and construction materials when infested at different stages. Termites may also create human health issues19.
The challenge of medication resistance gained by microbes and insect pests in today's pharmaceutical and agro-based sectors is difficult20, 21. As a result, both businesses should search for new cost effective antimicrobials and safe bio-pesticides. Synthetic pesticides are now available and have been proven to be infective and hinder non-target beneficial insects22. In recent years, biosurfactant research has expanded recently due to its use in various industrial activities. Biosurfactants are highly beneficial and vital in agriculture, soil remediation, oil recovery, bacterial and insect removal, and food sectors, among other things23, 24. Biosurfactants, or microbial surfactants, are biologically surface-active chemicals generated by microorganisms such as bacteria, yeast, and fungus in coastal habitats and oil-contaminated regions25, 26. Chemically manufactured surfactants and biosurfactants are the two types that are derived directly from natural settings27. Various biosurfactants are obtained from marine habitats28, 29. Consequently, scientists are looking for new biosurfactant manufacturing techniques based on naturally available bacteria30, 31. The advancement of such research demonstrates the importance of these biological compounds in environmental protection32. Bacillus, Pseudomonas, Rhodococcus, Alcaligenes, Corynebacterium and those bacterial genera are represented among the well-studied members23, 33.
Biosurfactants are extremely varied and have a wide range of uses34. These compounds' significant advantage is that some exhibit antibacterial, larvicidal, and insecticidal activities. This implies they may be used in the agricultural, chemical, pharmaceutical, and cosmetic sectors35,36,37,38. Since they are often biodegradable and ecologically beneficial, biosurfactants have been utilised in integrated pest control programmes to protect crops39. Therefore, to obtain fundamental knowledge on larvicidal and anti-termite activities of microbial biosurfactant produced by Enterobacter cloacae SJ2. We examined the mortality and histological changes at exposure to different concentrations of the rhamnolipid biosurfactant. In addition, we evaluate the widely used Quantitative Structure–Activity Relationship (QSAR) computer programme, Ecological Structure–Activity Relationship (ECOSAR) to derive the acute toxicity of microalgae, daphnids, and fish.
In the present study, the anti-termite activity (toxicity) of the purified biosurfactant at varying concentrations ranged from 30 to 50 mg/mL with an interval of 5 mg/mL was evaluated against the Indian white termites, O. obesus and the 4th instar larvae of Cx. quinquefasciatus mosquito larva. The 48 h LC50 concentration of the biosurfactant against the O. obesus and the Cx. quinquefasciatus. Mosquito larva was determined using the non-linear regression curve fit method. The results showed increased termite mortality with the increased concentration of the biosurfactant. Results showed larvicidal activity (Fig. 1) and anti-termite activity (Fig. 2) of biosurfactants with 48 h LC50 value (95% CI) of 26.49 mg/L (25.40 to 27.57) and 33.43 mg/L (31.09 to 35.68) respectively (Table 1). In terms of acute toxicity (48 h), biosurfactant belongs to the tested organisms’ “harmful” category. The biosurfactant produced in the present study showed excellent larvicidal activity with a 100% mortality rate within 24–48 h of exposure.
Calculation of LC50 values for larvicidal activity. Non-linear regression curve fit (solid line) and 95% confidence intervals (shaded area) on the relative mortality (%).
Calculation of LC50 values for anti-termite activity. Non-linear regression curve fit (solid line) and 95% confidence intervals (shaded area) on the relative mortality (%).
Under the microscope, morphological changes and abnormalities were observed at the end of the experiment. Control and treated groups at 40 × magnification showed morphologic changes. Most of the larvae treated with biosurfactant revealed growth disruption, as demonstrated in Fig. 3. Figure 3a displays a normal Cx. quinquefasciatus, and Fig. 3b shows abnormal Cx. quinquefasciatus larvae.
Effect of sub-lethal (LC50) dose of biosurfactant on Culex quinquefasciatus larval development. Light microscope images at 40 × magnification (a) Normal Cx. quinquefasciatus (b) Abnormal Cx. quinquefasciatus larvae.
In the present study, the histological examination of the treated larvae (Fig. 4) and termite (Fig. 5) revealed several abnormalities, including a reduction in the size of the abdominal area as well as damage to the muscles, epithelial layers, and midgut. Histology revealed the mechanism behind the inhibitory activity of biosurfactants used in the present study.
Histopathology of normal untreated 4th instar larvae of Cx. quinquefasciatus larva (Control: (a,b)) and treated with the biosurfactant (Treatment: (c,d)). Arrow indicates the treated gut epithelium (epi), nucleus (n) and muscles (mu). Bar = 50 µm.
Histopathology of normal untreated O. obesus (Control: (a,b)) and treated with the biosurfactant (Treatment: (c,d)). Arrow indicates the gut epithelium (epi) and muscles (mu) respectively. Bar = 50 µm.
The acute toxicity of rhamnolipid biosurfactant products top primary producer (green algae), primary consumer (daphnia) and secondary consumer (fish) were predicted in this work using ECOSAR. This programme employs sophisticated quantitative structure–activity connection models to assess toxicity based on molecular structure. Using structure activity relationship (SAR) software, the model calculates a substance's acute and long-term toxicity to aquatic species. In particular, Table 2. Summarizes several species’ estimated median lethal concentration (LC50) and median effective concentration (EC50). Globally Harmonized System of Classification and Labelling of Chemicals was used to classify the estimated toxicity into four levels (Table 3).
Controlling vector-borne diseases, especially from mosquitoes and strains like Ae. aegypti, is a difficult job nowadays40,41,42,43,44,45,46. While some chemically accessible pesticides, such as pyrethroids and organophosphates, are beneficial to some extent, they pose a major hazard to human health, including diabetes, reproductive diseases, neurological dysfunction, cancer, and respiratory illnesses, among other things4, 8, 47. Further, eventually, these insects develop resistance against them13, 43, 48. Thus, efficient and eco-friendly control measures from biological means will be a more welcome approach to mosquito control49, 50. Benelli51 suggest controlling mosquito vectors at the early instar stage will be more effective in urban areas, while they do not recommend larvicides in rural areas52. Tome et al.53 also proposed that controlling the mosquito at the immature stage would be a safe and easy strategy because they will be more sensitive to the control treatments54.
The biosurfactant production of the potent strain (E. cloacae SJ2) showed consistent and promising efficiency. In our previous study reported that the biosurfactant production by E. cloacae SJ2 was optimized using physicochemical parameters26. According to their study, the optimum condition of biosurfactant production for the potential isolate E. cloacae was 36 h incubation, 150 rpm agitation, pH 7.5, 37 °C, 1 ppt salinity, 2% glucose as carbon source and 1% yeast extract as nitrogen source, produced 2.61 g/L of biosurfactant. Furthermore, the biosurfactant properties were characterised using TLC, FTIR, and MALDI-TOF-MS. Which is confirmed that rhamnolipid biosurfactant. Glycolipid biosurfactants are among the most intensively studied classes among the other types biosurfactants55. They are comprised of carbohydrate and lipid moieties, mainly chains of fatty acids. Among the glycolipids, rhamno and sophoro lipids are the main representatives56. Rhamnolipid comprises two rhamnose sugar moieties linked to mono or di β-hydroxy decanoic acid57. Applications of rhamnolipids in medical and pharma industries are well known58, besides their use as a pesticide recently59.
The interaction of the biosurfactants with the respiratory syphon's hydrophobic area increases the larvae's exposure to the aqueous medium by allowing water to pass through their spiracular cavity. The biosurfactants’ presence also impacts tracheas, which have a range near the surface, making it easier for the larvae to climb to the surface for breathing. As a result, the water surface tension decreases. Because they cannot stick to the water’s surface, larvae drop to the bottom of the tank, which interferes with hydrostatic pressure, leading to excessive energy consumption and death by drowning38, 60. Ghribi61 reported similar results, that biosurfactant produced by Bacillus subtilis demonstrated larvicidal activity against Ephestia kuehniella. Similarly, the larvicidal activity of Cx. quinquefasciatus larvae by cyclic lipopeptide was also evaluated by Das and Mukherjee23.
The results of the present study on the larvicidal activity of a rhamnolipid biosurfactant against Cx. quinquefasciatus mosquito are supported by the findings of previous reports62, 63. For example, the application of surfactin-based biosurfactant produced from various bacteria belonging to Bacillus spp. and Pseudomonas spp. on mosquitocidal activity has been reported by several early reports64,65,66, mosquito larvicidal activity of a lipopeptide biosurfactant from B. subtilis23. Deepali et al.63 found a rhamnolipid biosurfactant at 10 mg/L isolated from Stenotrophomonas maltophilia with potent larvicidal activity. Silva et al.67 reported the larvicidal activity of a rhamnolipid biosurfactant at the concentration of 1 g/L against Ae. aegypti. Kanakdande et al.68 reported that lipopeptide biosurfactant produced by Bacillus subtilis results in total mortality of Culex larvae and termites with the lipophilic fractions of Eucalyptus. Similarly, Masendra et al.69 reported n-hexane and EtOAc lipophilic fractions of Eucalyptus pellita extract exhibit 61.7% mortality on worker termites (Cryptotermes cynocephalus Light.).
Parthipan et al.70 reported the insecticidal application of lipopeptide biosurfactants produced by B. subtilis A1 and Pseudomonas stutzeri NA3 against the malaria-causing Plasmodium parasite vector Anopheles stephensi. They observed longer pupal duration, shorter oviposition period, infertility, and reduced longevity of both larvae and pupae when treated with different concentrations of biosurfactant. The observed LC50 values for the biosurfactant from B. subtilis A1 were 3.58, 4.92, 5.37, 7.10 and 7.99 mg/L, respectively, for different states of larva (i.e.) larva I, II, III, IV and pupae stage. In contrast, it was 2.61, 3.68, 4.48, 5.55 and 6.99 mg/L for larva stages I-IV and pupae stage, respectively, for the biosurfactant of Pseudomonas stutzeri NA3. The delayed phenology of surviving larvae and pupa is thought to be the outcome of significant physiological metabolical abnormalities caused by the insecticidal treatments71.
The biosurfactant produced by Wickerhamomyces anomalus CCMA 0358 strain showed 100% larvicidal activity against Ae. aegypti in 24 h intervals38 than that reported by Silva et al. wherein the biosurfactant was produced from Pseudomonas aeruginosa using sunflower oil as a carbon source showed 100% elimination of the larvae in 48 h67. Abinaya et al.72 and Pradhan et al.73 have also confirmed the larvicidal action or insecticide of surfactants produced by several isolates of the genus Bacillus. A likely 100% mortality of mosquito larvae exposed to plant limnoids was reported in a previous research published by Senthil-Nathan et al.74.
The assessment of the sublethal effects of insecticides on insect biology is critical for integrated pest management programmes because sublethal doses/concentrations do not cause insect death but may reduce insect populations of future generations through interference with biological traits10. Siqueira et al.75 Observed a complete larvicidal activity (100% mortality) of a rhamnolipid biosurfactant (with 300 mg/ml) when tested at different concentrations ranging from 50 to 300 mg/mL against pyrethroid-resistant and susceptible Ae. aegypti strains at the larval stage. They analyzed the mortality time and the effects of sub lethal concentrations through the survival and swimming activity of the larvae. In addition, they observed reduced swimming rate at the sub-lethal concentrations of biosurfactants, such as with 50 mg/ml and 100 mg/mL, after 24–48 h of exposure. Toxicants exerting promising effects in sub-lethal effects are considered to be more effective in exerting multitude levels of damage to the exposed pests76.
Histological observation of our results revealed the biosurfactant produced by E. cloacae SJ2 significantly altered the tissues of mosquito larvae (Cx. quinquefasciatus) and termites (O. obesus). Similar kinds of abnormalities was caused by Ocimum kilimandscharicum oil formulation on An. gambiaes.s and An. Arabiensis was reported by Ochola77. Kamaraj et al.78 also describes identical morphological anomalies in An. stephensi larvae exposed to gold nanoparticles. Vasantha-Srinivasan et al.79 also reports that Piper beetle essential oil severely damaged the hut-lumen and epithelial layers of Ae. aegypti. Ragavendran et al. reported that, mosquito larvae treated with the 500 mg/mL mycelia extract of the indigenous fungus Penicillium sp. showed severe histological damage in Ae. aegypti and Cx. quinquefasciatus80. Earlier, Abinaya et al. examined the 4th instar larvae of An. stephensi and Ae. aegypti treated with Bacillus licheniformis exopolysaccharide discovered many histological alterations, including the stomach caeca, muscles shrinking, and injured and disordered nerve cord ganglia72. According to Ragavendran et al. after being treated with P. daleae mycelium extract, the midgut cells of the examined mosquitoes (4th instar larvae) exhibited swelling in the gut lumen, decreased intercellular contents, and nucleus degeneration81. Identical histological alterations were also observed in mosquito vector larvae treated with Acanthospermum hispidum leaf extracts indicating insecticidal potentials of treated compounds50.
Internationally, the application of ECOSAR software has been acknowledged82. According to present study, ECOSAR acute toxicity of biosurfactants to microalgae (C. vulgaris), fish and daphnid (D. magna) belong to the category of “toxic” as defined by the United Nations83. The ECOSAR eco-toxicity model uses SARs and QSARs to forecast substances’ acute and long-term toxicity, and it is often used to predict the toxicity of organic pollutants82, 84.
Paraformaldehyde, Sodium Phosphate Buffer (pH 7.4), and all other chemicals used in this study were purchased from HiMedia Laboratories, India.
Biosurfactant production was carried out in a 500 ml conical flask containing 200 ml of sterile Bushnell Haas medium supplemented with 1% crude oil as the sole carbon source. The pre-culture of Enterobacter cloacae SJ2 was inoculated (1.4 × 104 CFU/mL) and incubated at 37 °C in an orbital shaker at 200 rpm for seven days. After the incubation period, the biosurfactant was extracted by centrifugation of culture medium at 4 °C for 20 min at 3400×g, and the resultant supernatant was utilized for screening purposes. Biosurfactant optimization and characterization procedures were adopted from our earlier studies26.
The larvae of Culex quinquefasciatus was were obtained from Centre of Advanced Study (CAS) in Marine Biology, Parangipettai, Tamil Nadu (India). The larvae were kept in plastic containers having deionized water and were maintained at 27 ± 2 °C under and a photoperiod of 12:12 (light: dark). The mosquito larvae were fed with 10% glucose solution.
Culex quinquefasciatus larvae were found in the septic tanks that were left open and unprotected. A standard classification manual was used to identify and raise the larvae in the laboratory85.The WHO recommendations were followed for conducting the larvicidal test86. Cx. quinquefasciatus larvae of the fourth instar were collected in three sets of 25 numbers in 50 mL of water in a closed test tube with an air gap of two-thirds of its capacity. Biosurfactant (0–50 mg/mL) was applied to each tube individually and kept at 25 °C. Only distilled water (50 mL) was used in the control tube. A dead larva was considered to be one that showed no signs of swimming throughout the incubation phase (12–48 h)87. The percentage of larval mortality was calculated using Eq. (1)88.
The family Odontotermitidae includes the Indian white termite, Odontotermes obesus, found in the rotting logs at Agriculture Campus (Annamalai University, India). This biosurfactant (0–50 mg/mL) was tested to see whether it was hazardous using the usual procedure. After drying in a laminar airflow for 30 min, each strip of Whatman paper was coated with concentrations of 30, 40, or 50 mg/mL of biosurfactant. Pre-coated and uncoated paper strips were tested and compared in the centre of the Petri dish. There were around thirty active O. obesus termites in each Petri dish. The control and test termites received wet paper as a food source. All the Petri dishes were kept at room temperature throughout the incubation period. After 12, 24, 36, and 48 h, the termites had died off89, 90. Termite mortality percentages at various biosurfactant concentrations were then estimated using Eq. (2).
The samples kept on ice and packed in microtubes containing 100 mL of 0.1 M sodium phosphate buffer (pH 7.4) were sent to the Histology Laboratory of the Central Aquaculture Pathology Laboratory (CAPL) at Rajiv Gandhi Centre for Aquaculture (RGCA), Sirkali, Mayiladuthurai District, Tamil Nadu, India for further analysis. The sample was immediately fixed in 4% paraformaldehyde for 48 h at 37 °C.
After the fixing phase, the material was washed thrice with 0.1 M sodium phosphate buffer, pH 7.4, dehydrated in an ascending series of ethyl alcohol, and soaked in LEICA resin for seven days. After that, the substance was placed in plastic moulds filled with resin and polymerizer and then in an oven set at 37 °C until the blocks containing the substance were fully polymerized.
Following polymerization, the blocks were sectioned in a LEICA RM2235 Microtome (Rankin Biomedical Corporation 10,399 Enterprise Dr. Davisburg, MI 48,350, United States) with a thickness of 3 mm. The portions were gathered on glass slides, six parts per slide. The slides were dried at room temperature before being stained with hematoxylin for 7 min and washed for 4 min in running water. In addition, the eosin solution was applied to the skin for 5 min and washed for 5 min with running water.
Aquatic organisms from various tropical levels were used for predicting acute toxicity: 96 h fish LC50, 48 h D. magna LC50 and 96 h green algae EC50. The toxicity assessment of rhamnolipid biosurfactant for fish and green algae was carried out using ECOSAR software for Windows, Version 2.2, developed by US EPA. (Available online at https://www.epa.gov/tsca-screening-tools/ecological-structure-activity-relationships-ecosar-predictive-model).
All larvicidal and anti-termite activity tests were performed in triplicate. The larval and termite mortality data were subjected to non-linear regression (log dose–response variable parameter) to calculate the median lethal concentration (LC50) with 95% confidence limits to produce concentration–response curves using Prism® (Version 8.0, GraphPad Software Inc., USA)84, 91.
The current research reveals the potential of the microbial biosurfactant generated by E. cloacae SJ2 as a possible mosquito larvicidal and anti-termite agent, and this work will lead to a better understanding of the mechanism behind the larvicidal and anti-termite action. Histological study of larvae treated with biosurfactant showed damage in the digestive tract, midgut, cortex, and hyperplasia of gut epithelial cells. The results revealed toxicological evaluation of rhamnolipid biosurfactant produced by E. cloacae SJ2 using anti-termite and larvicidal activity revealed that this isolate was a potential biological insecticide controlling mosquito (Cx. quinquefasciatus), and termite (O. obesus) vector-borne diseases. It is necessary to understand the basic environmental toxicity of biosurfactants and their potential impacts on the environment. This study provides a scientific basis for the environmental risk assessment of biosurfactants.
All data generated or analysed during this study are included in this published article.
Vivekanandhan, P. et al. Comparative analysis of major mosquito vectors response to seed-derived essential oil and seed pod-derived extract from Acacia nilotica. Int. J. Environ. Res. Public Health 15, 388 (2018).
PubMed PubMed Central Google Scholar
Jones, R. T., Ant, T. H., Cameron, M. M. & Logan, J. G. Novel control strategies for mosquito-borne diseases. Philos. Trans. R. Soc. B Biol. Sci. 376, 20190802 (2021).
Kumar, K. K. et al. Microbial biopesticides for insect pest management in India: Current status and future prospects. J. Invertebr. Pathol. 165, 74–81 (2019).
Thanigaivel, A. et al. Impact of Terminalia chebula Retz. against Aedes aegypti L. and non-target aquatic predatory insects. Ecotoxicol. Environ. Saf. 137, 210–217 (2017).
Allan, R., Budge, S. & Sauskojus, H. What sounds like Aedes, acts like Aedes, but is not Aedes? Lessons from dengue virus control for the management of invasive Anopheles. Lancet Glob. Health 11, e165–e169 (2023).
Hussni Hasan, N. R. et al. From the sea to mosquito control: The potential of Halymenia dilatata marine alga as an eco-friendly mosquitocidal agent. Sustainability 15, 11900 (2023).
Falqueto, S. A. et al. Bacillus spp. metabolites are effective in eradicating Aedes aegypti (Diptera: Culicidae) larvae with low toxicity to non-target species. J. Invertebr. Pathol. 179, 107525 (2021).
Priya, S. S. et al. Bioactive molecules derived from plants in managing dengue vector Aedes aegypti (Linn.). Molecules 28, 2386 (2023).
CAS PubMed PubMed Central Google Scholar
McGraw, E. A. & O’Neill, S. L. Beyond insecticides: New thinking on an ancient problem. Nat. Rev. Microbiol. 11, 181–193 (2013).
Vasantha-Srinivasan, P. et al.Aspergillus flavus (Link) toxins reduce the fitness of dengue vector Aedes aegypti (Linn.) and their non-target toxicity against aquatic predator.Microb.Pathog.128, 281–287 (2019).
Martheswaran, T. K., Hamdi, H., Al-Barty, A., Zaid, A. A. & Das, B. Prediction of dengue fever outbreaks using climate variability and Markov chain Monte Carlo techniques in a stochastic susceptible-infected-removed model. Sci. Rep. 12, 5459 (2022).
CAS ADS PubMed PubMed Central Google Scholar
Mustafa, M. S., Rasotgi, V., Jain, S. & Gupta, V. Discovery of fifth serotype of dengue virus (DENV-5): A new public health dilemma in dengue control. Med. J. Armed Forces India 71, 67–70 (2015).
Thanigaivel, A. et al. Toxic effect of essential oil and its compounds isolated from Sphaeranthus amaranthoides Burm. F. against dengue mosquito vector Aedes aegypti Linn. Pestic. Biochem. Physiol. 160, 163–170 (2019).
Thanigaivel, A. et al. Chemicals isolated from Justicia adhatoda Linn reduce fitness of the mosquito, Aedes aegypti L. Arch. Insect Biochem. Physiol. 94, e21384 (2017).
Radhakrishnan, N. et al. Chemical screening and mosquitocidal activity of essential oil derived from Mikania scandens (L.) Willd. against Anopheles gambiae Giles and their non-toxicity on mosquito predators. Life 16, 2169959 (2023).
Fitzgibbon, W., Morgan, J., Webb, G. & Wu, Y. Maritime transport and the threat of bio invasion and the spread of infectious disease. In Computation and Big Data for Transport Vol. 54 (eds Diez, P. et al.) 69–73 (Springer International Publishing, 2020).
Chouvenc, T., Šobotník, J., Engel, M. S. & Bourguignon, T. Termite evolution: Mutualistic associations, key innovations, and the rise of Termitidae. Cell. Mol. Life Sci. 78, 2749–2769 (2021).
Devi, K. K., Seth, N., Kothamasi, S. & Kothamasi, D. Hydrogen cyanide-producing Rhizobacteria kill subterranean termite Odontotermes obesus (Rambur) by cyanide poisoning under in vitro conditions. Curr. Microbiol. 54, 74–78 (2007).
Mitchell, S. J. & Silver, R. A. GABA spillover from single inhibitory axons suppresses low-frequency excitatory transmission at the cerebellar glomerulus. J. Neurosci. 20, 8651–8658 (2000).
CAS PubMed PubMed Central Google Scholar
Senthil-Nathan, S. A review of biopesticides and their mode of action against insect pests. In Environmental Sustainability (eds Thangavel, P. & Sridevi, G.) 49–63 (Springer India, 2015). https://doi.org/10.1007/978-81-322-2056-5_3.
Senthil-Nathan, S. A review of resistance mechanisms of synthetic insecticides and botanicals, phytochemicals, and essential oils as alternative larvicidal agents against mosquitoes. Front. Physiol. 10, 1591 (2020).
PubMed PubMed Central Google Scholar
Parewa, H. P. et al. Role of biofertilizers and biopesticides in organic farming. In Advances in Organic Farming (eds Parewa, H. P. et al.) 133–159 (Elsevier, 2021). https://doi.org/10.1016/B978-0-12-822358-1.00009-2.
Das, K. & Mukherjee, A. K. Assessment of mosquito larvicidal potency of cyclic lipopeptides produced by Bacillus subtilis strains. Acta Trop. 97, 168–173 (2006).
Rawat, G., Choudhary, R., Kumar, V. & Kumar, V. Biosurfactants: Promising biomolecules for environmental cleanup. In Sustainable Solutions for Environmental Pollution (ed. El-Gendy, N. S.) 293–319 (Wiley, 2022). https://doi.org/10.1002/9781119827665.ch6.
Shekhar, S., Sundaramanickam, A. & Balasubramanian, T. Biosurfactant producing microbes and their potential applications: A review. Crit. Rev. Environ. Sci. Technol. 45, 1522–1554 (2015).
Harikrishnan, S. et al. Production and characterization of biosurfactant from Enterobacter cloacae SJ2 isolated from marine sponge Clathria sp. Biomass Convers. Biorefin. https://doi.org/10.1007/s13399-022-03466-1 (2022).
Anna, J. P. & Parthasarathi, R. Production and characterization of biosurfactant from Pseudomonas aeruginosa PBSC1 isolated from mangrove ecosystem. Afr. J. Biotechnol. 13, 3394–3401 (2014).
Gudiña, E., Teixeira, J. & Rodrigues, L. Biosurfactants produced by marine microorganisms with therapeutic applications. Mar. Drugs 14, 38 (2016).
PubMed PubMed Central Google Scholar
Kubicki, S. et al. Marine biosurfactants: Biosynthesis, structural diversity and biotechnological applications. Mar. Drugs 17, 408 (2019).
CAS PubMed PubMed Central Google Scholar
Cameotra, S. S. & Makkar, R. S. Biosurfactant-enhanced bioremediation of hydrophobic pollutants. Pure Appl. Chem. 82, 97–116 (2010).
Abbasian, F. et al. Microbial diversity and hydrocarbon degrading gene capacity of a crude oil field soil as determined by metagenomics analysis. Biotechnol. Prog. 32, 638–648 (2016).
Diab, A., Sami, S. & Diab, A. Production, characterization and application of a new biosurfactant derived from egyptian sunflower seeds. Int. J. Sci. Res. 5, 602–612 (2016).
Eras-Muñoz, E., Farré, A., Sánchez, A., Font, X. & Gea, T. Microbial biosurfactants: A review of recent environmental applications.Bioengineered 13, 12365–12391 (2022).
PubMed PubMed Central Google Scholar
Vieira, I. M. M., Santos, B. L. P., Ruzene, D. S. & Silva, D. P. An overview of current research and developments in biosurfactants. J. Ind. Eng. Chem. 100, 1–18 (2021).
Kim, S. K. et al. Insecticidal activity of rhamnolipid isolated from Pseudomonas sp. EP-3 against green peach aphid (Myzus persicae). J. Agric. Food Chem. 59, 934–938 (2011).
Gomaa, E. Z. Antimicrobial activity of a biosurfactant produced by Bacillus licheniformis strain M104 grown on whey. Braz. Arch. Biol. Technol. 56, 259–268 (2013).
Jemil, N., Ben Ayed, H., Manresa, A., Nasri, M. & Hmidet, N. Antioxidant properties, antimicrobial and anti-adhesive activities of DCS1 lipopeptides from Bacillus methylotrophicus DCS1. BMC Microbiol. 17, 144 (2017).
PubMed PubMed Central Google Scholar
de Andrade Teixeira Fernandes, N. et al. Eco-friendly biosurfactant from Wickerhamomyces anomalus CCMA 0358 as larvicidal and antimicrobial. Microbiol. Res. 241, 126571 (2020).
Yang, S. Y. et al. Characterization of biosurfactants as insecticidal metabolites produced by Bacillus subtilis Y9: Aphicidal surfactin isomers. Entomol. Res. 47, 55–59 (2017).
Harris, A. F., Rajatileka, S. & Ranson, H. Pyrethroid resistance in Aedes aegypti from Grand Cayman. Am. J. Trop. Med. Hyg. 83, 277–284 (2010).
CAS PubMed PubMed Central Google Scholar
Demok, S. et al. Insecticide resistance status of Aedes aegypti and Aedes albopictus mosquitoes in Papua New Guinea. Parasit. Vectors 12, 333 (2019).
CAS PubMed PubMed Central Google Scholar
Sá, E. L. R. D. et al. Evaluation of insecticide resistance in Aedes aegypti populations connected by roads and rivers: The case of Tocantins state in Brazil. Mem. Inst. Oswaldo Cruz 114, e180318 (2019).
PubMed PubMed Central Google Scholar
Gunathilaka, N. et al. Use of mechanical and behavioural methods to eliminate female Aedes aegypti and Aedes albopictus for sterile insect technique and incompatible insect technique applications. Parasit. Vectors 12, 148 (2019).
PubMed PubMed Central Google Scholar
Ramos-Nino, M. E. et al. High prevalence of Phasi Charoen-like virus from wild-caught Aedes aegypti in Grenada, W.I. as revealed by metagenomic analysis. PLoS One 15, e0227998 (2020).
CAS PubMed PubMed Central Google Scholar
Jinu, U., Rajakumaran, S., Senthil-Nathan, S., Geetha, N. & Venkatachalam, P. Potential larvicidal activity of silver nanohybrids synthesized using leaf extracts of Cleistanthus collinus (Roxb.) Benth. ex Hook.f. and Strychnos nux-vomica L. nux-vomica against dengue, Chikungunya and Zika vectors. Physiol. Mol. Plant Pathol. 101, 163–171 (2018).
Chellappandian, M. et al.Effect of target and non-target botanical pesticides on Trichodesma indicum (Linn) R. Br.and their chemical derivatives against the dengue vector, Aedes aegypti L.. Environ.Sci.Pollut.Res.26, 16303–16315 (2019).
Rani, L. et al. An extensive review on the consequences of chemical pesticides on human health and environment. J. Clean. Prod. 283, 124657 (2021).
Paiva, DR et al.A potent larvicidal agent against Aedes aegypti mosquito from cardanol.An. Acad.Brazil.Science 89, 373–382 (2017).
Benelli, G. et al. Mosquito control with green nanopesticides: Towards the one health approach? A review of non-target effects. Environ. Sci. Pollut. Res. 25, 10184–10206 (2018).
Vivekanandhan, P., Senthil-Nathan, S. & Shivakumar, M. S. Larvicidal, pupicidal and adult smoke toxic effects of Acanthospermum hispidum (DC) leaf crude extracts against mosquito vectors. Physiol. Mol. Plant Pathol. 101, 156–162 (2018).
Benelli, G. Research in mosquito control: Current challenges for a brighter future. Parasitol. Res. 114, 2801–2805 (2015).
Benelli, G. & Beier, J. C. Current vector control challenges in the fight against malaria. Acta Trop. 174, 91–96 (2017).
Tomé, H. V., Pascini, T. V., Dângelo, R. A., Guedes, R. N. & Martins, G. F. Survival and swimming behavior of insecticide-exposed larvae and pupae of the yellow fever mosquito Aedes aegypti. Parasit. Vectors 7, 195 (2014).
PubMed PubMed Central Google Scholar
Lee, S. J., Kim, J. H. & Lee, S. C. Effects of oil-film layer and surfactant on the siphonal respiration and survivorship in the fourth instar larvae of Aedes togoi mosquito in laboratory conditions. Sci. Rep. 8, 5694 (2018).
ADS PubMed PubMed Central Google Scholar
Adu, S. A., Twigg, M. S., Naughton, P. J., Marchant, R. & Banat, I. M. Characterisation of cytotoxicity and immunomodulatory effects of glycolipid biosurfactants on human keratinocytes. Appl. Microbiol. Biotechnol. 107, 137–152 (2023).
Shao, B. et al. Effects of rhamnolipids on microorganism characteristics and applications in composting: A review. Microbiol. Res. 200, 33–44 (2017).
CAS ADS PubMed Google Scholar
Correia, J., Gudiña, E. J., Lazar, Z., Janek, T. & Teixeira, J. A. Cost-effective rhamnolipid production by Burkholderia thailandensis E264 using agro-industrial residues. Appl. Microbiol. Biotechnol. 106, 7477–7489 (2022).
Jadhav, J. V., Anbu, P., Yadav, S., Pratap, A. P. & Kale, S. B. Sunflower acid oil-based production of rhamnolipid using Pseudomonas aeruginosa and its application in liquid detergents. J. Surfactants Deterg. 22, 463–476 (2019).
Sachdev, D. P. & Cameotra, S. S. Biosurfactants in agriculture. Appl. Microbiol. Biotechnol. 97, 1005–1016 (2013).
CAS PubMed PubMed Central Google Scholar
Christophers, S. Aëdes aegypti (L.) the yellow fever mosquito: Its life history, bionomics and structure. Science 133, 1473–1474 (1960).
Ghribi, D. et al. Investigation of antimicrobial activity and statistical optimization of Bacillus subtilis SPB1 biosurfactant production in solid-state fermentation. J. Biomed. Biotechnol. 2012, 1–12 (2012).
Govindasamy, B. et al. Evaluation of Salmonella bongori derived biosurfactants and its extracellular protein separation by SDS-PAGE using petridishes: A simply modified approach. Int. J. Biol. Macromol. 140, 156–167 (2019).
Korade, D. & Sneha, P. Larvicidal activity of rhamnolipid biosurfactant produced by Stenotrophomonas maltophilia. Int. J. Sci. Eng. Res. 5, 60–63 (2014).
Geetha, I. & Manonmani, A. M. Surfactin: A novel mosquitocidal biosurfactant produced by Bacillus subtilis ssp. subtilis (VCRC B471) and influence of abiotic factors on its pupicidal efficacy: Abiotic factors and mosquitocidal efficacy of surfactin. Lett. Appl. Microbiol. 51, 406–412 (2010).
Geetha, I., Manonmani, A. M. & Paily, K. P. Identification and characterization of a mosquito pupicidal metabolite of a Bacillus subtilis subsp. subtilis strain. Appl. Microbiol. Biotechnol. 86, 1737–1744 (2010).
Revathi, K. et al.Effects of Bacillus subtilis metabolites on larval Aedes aegypti L. Pestic.Biochem.Physiol.107, 369–376 (2013).
Silva, V. L., Lovaglio, R. B., Von Zuben, C. J. & Contiero, J. Rhamnolipids: Solution against Aedes aegypti?. Front. Microbiol. https://doi.org/10.3389/fmicb.2015.00088 (2015).
Article PubMed PubMed Central Google Scholar
Kanakdande, A. P. & Khobragade, C. N. Production of biosurfactant from Bacillus subtilis (MF 582633) and its evaluation for antimicrobial, antioxidant, larvicidal and antitermite activities. IJCT, 261 (2019).
Masendra, Purba, B. A. V. & Lukmandaru, G. Anti-termite activity from the lipophilic fractions of Eucalyptus pellita F. Muell heartwood. J. Indian Acad. Wood Sci. 18, 52–58 (2021).
Parthipan, P. et al. Biosurfactants produced by Bacillus subtilis A1 and Pseudomonas stutzeri NA3 reduce longevity and fecundity of Anopheles stephensi and show high toxicity against young instars. Environ. Sci. Pollut. Res. 25, 10471–10481 (2018).
Senthil-Nathan, S. et al. Efficacy of Melia azedarach L. extract on the malarial vector Anopheles stephensi Liston (Diptera: Culicidae). Bioresour. Technol. 97, 1316–1323 (2006).
Abinaya, M. et al. Structural characterization of Bacillus licheniformis Dahb1 exopolysaccharide—Antimicrobial potential and larvicidal activity on malaria and Zika virus mosquito vectors. Environ. Sci. Pollut. Res. 25, 18604–18619 (2018).
Pradhan, A. K. et al. Cyclic lipopeptide biosurfactant from Bacillus tequilensis exhibits multifarious activity. 3 Biotech 8, 261 (2018).
PubMed PubMed Central Google Scholar
Senthil-Nathan, S., Kalaivani, K. & Murugan, K. Effects of neem limonoids on the malaria vector Anopheles stephensi Liston (Diptera: Culicidae).Acta Trop.96, 47–55 (2005).
De Paula Siqueira, T. et al. Rhamnolipids on Aedes aegypti larvae: A potential weapon against resistance selection. 3 Biotech 11, 172 (2021).
PubMed PubMed Central Google Scholar
Karthi, S. et al. Biological effects of Avicennia marina (Forssk.) vierh. extracts on physiological, biochemical, and antimicrobial activities against three challenging mosquito vectors and microbial pathogens. Environ. Sci. Pollut. Res. 27, 15174–15187 (2020).
Ochola, J. B. et al. Mosquitoes larvicidal activity of Ocimum kilimandscharicum oil formulation under laboratory and field-simulated conditions. Insects 13, 203 (2022).
PubMed PubMed Central Google Scholar
Kamaraj, C. et al. Green synthesis of gold nanoparticles using Gracilaria crassa leaf extract and their ecotoxicological potential: Issues to be considered. Environ. Res. 213, 113711 (2022).
Vasantha-Srinivasan, P. et al. Comparative analysis of mosquito (Diptera: Culicidae: Aedes aegypti Liston) responses to the insecticide Temephos and plant derived essential oil derived from Piper betle L. Ecotoxicol. Environ. Saf. 139, 439–446 (2017).
Ragavendran, C. et al. Larvicidal, Histopathological, antibacterial activity of indigenous fungus Penicillium sp. against Aedes aegypti L and Culex quinquefasciatus (say) (Diptera: Culicidae) and its acetylcholinesterase inhibition and toxicity assessment of Zebrafish (Danio rerio). Front. Microbiol. 10, 427 (2019).
PubMed PubMed Central Google Scholar
Ragavendran, C., Mariappan, T. & Natarajan, D. Larvicidal, histopathological efficacy of Penicillium daleae against larvae of Culex quinquefasciatus and Aedes aegypti plus biotoxicity on Artemia nauplii a non-target aquatic organism. Front. Pharmacol. 8, 773 (2017).
CAS PubMed PubMed Central Google Scholar
Li, C. et al. Investigation of the transformation and toxicity of trichlorfon at the molecular level during enzymic hydrolysis of apple juice. Food Chem. 344, 128653 (2021).
United Nations Economic Commission for Europe Secretariat. Globally harmonized system of classification and labelling of chemicals (GHS) (United Nations New York, 2011).
Sudarshan, S., Bharti, V. S., Harikrishnan, S., Shukla, S. P. & RathiBhuvaneswari, G. Eco-toxicological effect of a commercial dye Rhodamine B on freshwater microalgae Chlorella vulgaris. Arch. Microbiol. 204, 658 (2022).
Cutwa, M. M. & Turingan, R. G. Intralocality variation in feeding biomechanics and prey use in Archosargus probatocephalus (Teleostei, Sparidae), with implications for the ecomorphology of fishes. Environ. Biol. Fish. 59, 191–198 (2000).
World Health Organization. Guidelines for laboratory and field testing of mosquito larvicides. https://apps.who.int/iris/handle/10665/69101 (2005).
Amala, K. et al. Bioefficacy of Epaltes divaricata (L.) n-hexane extracts and their major metabolites against the Lepidopteran Pests Spodoptera litura (fab.) and dengue mosquito Aedes aegypti (Linn.). Molecules 26, 3695 (2021).
CAS PubMed PubMed Central Google Scholar
Abbott, W. S. A method of computing the effectiveness of an insecticide. 1925. J. Am. Mosq. Control Assoc. 3, 302–303 (1987).
Sharma, S. & Vredenburg, H. Proactive corporate environmental strategy and the development of competitively valuable organizational capabilities. Strateg. Manag. J. 19, 729–753 (1998).
Roszaini, K., Nor Azah, M. A., Mailina, J., Zaini, S. & Mohammad Faridz, Z. Toxicity and antitermite activity of the essential oils from Cinnamomum camphora, Cymbopogon nardus, Melaleuca cajuputi and Dipterocarpus sp. against Coptotermes curvignathus. Wood Sci. Technol. 47, 1273–1284 (2013).
Vereecken, S., Vanslembrouck, A., Kramer, I. M. & Müller, R. Phenotypic insecticide resistance status of the Culex pipiens complex: A European perspective. Parasit. Vectors 15, 423 (2022).
CAS PubMed PubMed Central Google Scholar
The author gratefully thanks to Rashtriya Uchchatar Shiksha Abhiyan (RUSA) 2.0/ Filed- 5 (Marine Ecosystem Assessment) grant and Annamalai University, Tamil Nadu, India, for providing lab facilities. The author acknowledges King Saud University, Riyadh, Saudi Arabia, for providing the Researchers Supporting Project (RSP-2023R414).
The authors appreciate the Researchers supporting project number (RSP-2023R414), King Saud University, Riyadh, Saudi Arabia.
Centre of Advanced Study in Marine Biology, Faculty of Marine Sciences, Annamalai University, Parangipettai, Tamil Nadu, 608502, India
Sekar Harikrishnan & Singaram Jayalakshmi
Department of Aquatic Environment Management, TNJFU- Dr. M.G.R Fisheries College and Research Institute, Thalainayeru, Tamil Nadu, 614712, India
Department of Microbiology, Faculty of Science, Annamalai University, Annamalai Nagar, Chidambaram, Tamil Nadu, India
Department of Microbiology, Sree Balaji Medical College and Hospital, Chennai, Tamil Nadu, India
Department of Environmental & Water Resources Engineering, School of Civil Engineering (SCE), Vellore Institute of Technology, Vellore, Tamil Nadu, 632014, India
Department of Medical Sciences, University College of MAIWP International, Taman Batu Muda, 68100, Batu Caves, Kuala Lumpur, Malaysia
Department of Conservative Dentistry and Endodontics, Saveetha Dental College and Hospitals, Saveetha Institute of Medical and Technical Sciences, Saveetha University, Chennai, India
Department of Zoology, College of Science, King Saud University, 11451, Riyadh, Saudi Arabia
School of Chemical Engineering, Chonnam National University, Gwangju, South Korea
Environmental Molecular Microbiology Research Laboratory, Department of Biotechnology, Thiruvalluvar University, Serkkadu, Vellore, Tamil Nadu, 632115, India
You can also search for this author in PubMed Google Scholar
You can also search for this author in PubMed Google Scholar
You can also search for this author in PubMed Google Scholar
You can also search for this author in PubMed Google Scholar
You can also search for this author in PubMed Google Scholar
You can also search for this author in PubMed Google Scholar
You can also search for this author in PubMed Google Scholar
You can also search for this author in PubMed Google Scholar
You can also search for this author in PubMed Google Scholar
You can also search for this author in PubMed Google Scholar
S.H. Conceptualization; Data curation; Formal analysis; Writing—original draft. S.S. Methodology, Software, Writing—review & editing. K.S. Methodology, Investigation, Writing—review & editing. M.S.N. Resources; Writing—review & editing. J.N. Methodology, Software, Writing—review & editing. V.R. Resources; Writing—review & editing. B.O.A. Resources; Writing—review & editing. P.A. Funding acquisition; Resources; Writing—review & editing. A.R. Validation; Writing-introduction & editing. S.J. Resources; Methodology; Validation; Writing—review & editing.
Correspondence to Sekar Harikrishnan or Jayaraman Narenkumar.
The authors declare no competing interests.
Springer Nature remains neutral with regard to jurisdictional claims in published maps and institutional affiliations.
Open Access This article is licensed under a Creative Commons Attribution 4.0 International License, which permits use, sharing, adaptation, distribution and reproduction in any medium or format, as long as you give appropriate credit to the original author(s) and the source, provide a link to the Creative Commons licence, and indicate if changes were made. The images or other third party material in this article are included in the article's Creative Commons licence, unless indicated otherwise in a credit line to the material. If material is not included in the article's Creative Commons licence and your intended use is not permitted by statutory regulation or exceeds the permitted use, you will need to obtain permission directly from the copyright holder. To view a copy of this licence, visit http://creativecommons.org/licenses/by/4.0/.
Harikrishnan, S., Sudarshan, S., Sivasubramani, K. et al. Larvicidal and anti-termite activities of microbial biosurfactant produced by Enterobacter cloacae SJ2 isolated from marine sponge Clathria sp.. Sci Rep 13, 15153 (2023). https://doi.org/10.1038/s41598-023-42475-6
DOI: https://doi.org/10.1038/s41598-023-42475-6
Anyone you share the following link with will be able to read this content:
Sorry, a shareable link is not currently available for this article.
Provided by the Springer Nature SharedIt content-sharing initiative
By submitting a comment you agree to abide by our Terms and Community Guidelines. If you find something abusive or that does not comply with our terms or guidelines please flag it as inappropriate.
Scientific Reports (Sci Rep) ISSN 2045-2322 (online)
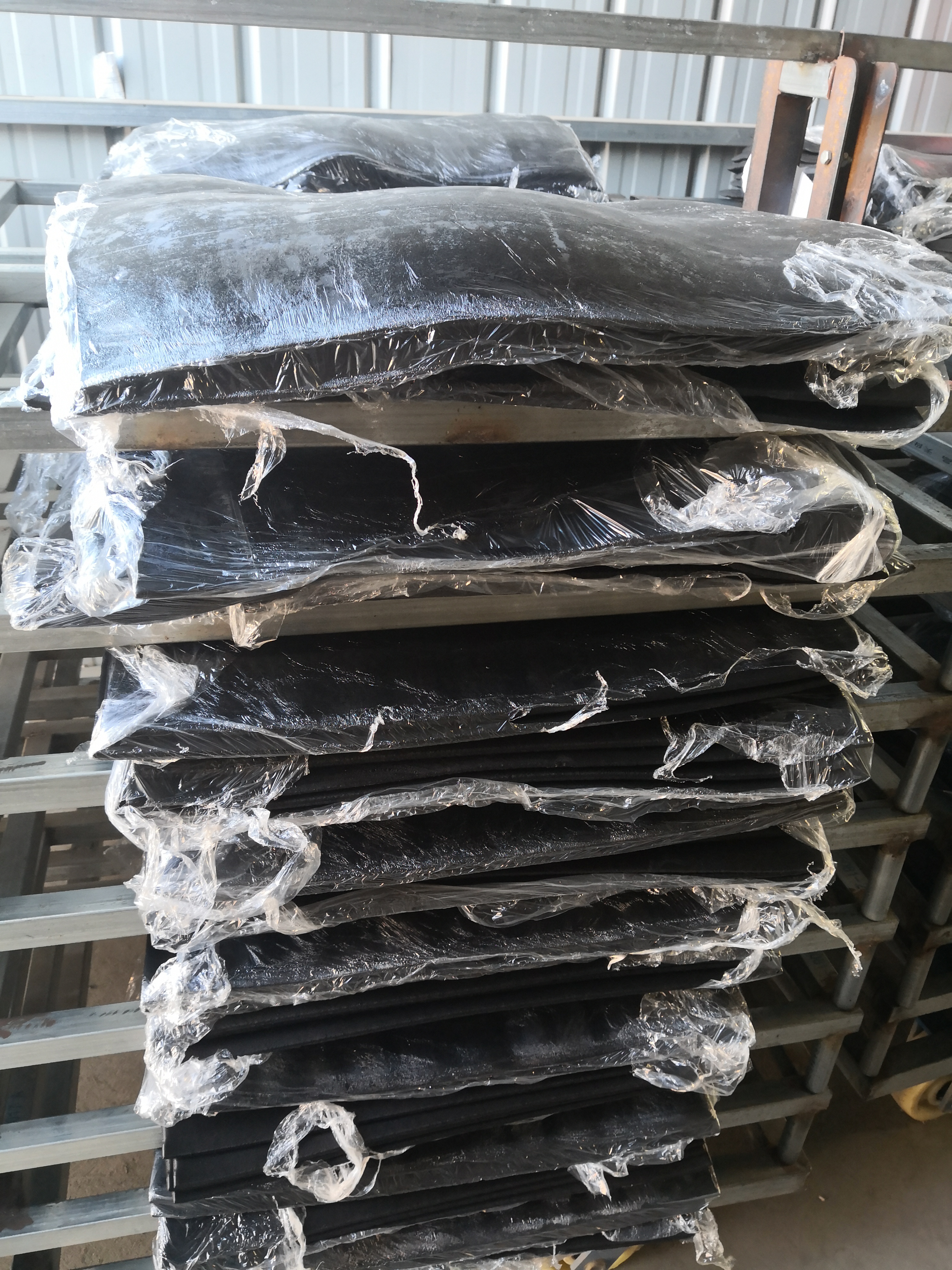
Phenylglycine Sign up for the Nature Briefing newsletter — what matters in science, free to your inbox daily.